The known: Early diagnosis of systemic envenoming following snakebite is important for early antivenom treatment. The blood concentration of D‐dimer is elevated in patients with venom‐induced consumption coagulopathy, the most frequent systemic envenoming syndrome.
The new: A D‐dimer level exceeding 2.5 mg/L within six hours of snakebite was diagnostic of venom‐induced consumption coagulopathy, with sensitivity and specificity each greater than 90%.
The implications: Quantitative D‐dimer assessment 2–6 hours after snakebite could aid early diagnosis of snake envenoming with venom‐induced consumption coagulopathy.
Snake envenoming, an uncommon but severe medical condition, is a neglected tropical disease.1 Some of the most dangerous snakes in the world are native to Australia, where snakebites cause deaths2 and thrombotic microangiopathy and acute kidney injury that may lead to chronic kidney disease.3 The annual number of snakebite deaths has not changed in Australia over the past 40years,4,5 despite the availability of safe and efficacious antivenoms and effective intensive care.
Antivenom is the only specific therapy for treating snake envenoming,6 and providing it early effectively prevents myotoxicity and neurotoxicity.7,8,9 Administering antivenom within four hours of snakebite reduces the likelihood of neurotoxicity and need for intubation;7,10 provided within 3–6 hours, depending on the snake species, it reduces the likelihood of myotoxicity.7,8,11 Despite the effectiveness of early antivenom, the median time to first administration had not declined during 2005–2015.2 New approaches to the early diagnosis of systemic envenoming are needed to reduce the number of deaths and the morbidity related to delayed complications.
Venom‐induced consumption coagulopathy (VICC) is the most frequent systemic effect of envenoming by Australian snakes (about three‐quarters of cases).2 For this reason, coagulation studies are routinely undertaken in patients after snakebite, including determination of prothrombin time or international normalised ratio (INR), activated partial thromboplastin time (aPTT), and fibrinogen and D‐dimer levels. VICC is an early manifestation of systemic envenoming, developing within hours;12 coagulation study findings are typically abnormal within two hours.13
The increasing availability of D‐dimer testing for diagnosing thrombo‐embolic disease14 provides an opportunity for even earlier diagnosis of VICC. Blood levels of D‐dimer, a fibrin degradation product, are extremely sensitive to activation of the clotting pathway, and its elevated concentration is the earliest positive coagulation test marker for VICC. More rapid diagnosis of VICC would facilitate earlier antivenom treatment and potentially reduce the risk of later neurotoxicity and myotoxicity in people with bites by snakes that cause VICC.
We therefore examined the accuracy and marginal value of quantitative D‐dimer testing for diagnosing VICC in a retrospective analysis of a large database of envenomings by Australian snakes.
Methods
To determine whether elevated D‐dimer concentration might serve as an early diagnostic marker of VICC, we reviewed data for snakebite cases collected prospectively by the Australian Snakebite Project (ASP).2 The ASP recruits people with suspected or confirmed snakebite at more than 200 hospitals across Australia. Cases are notified to the ASP by local hospital investigators, by the National Poisons Information Centre Network, directly to ASP investigators via a national free call number (1800 676944), and by clinical toxicologists. ASP coordinators fax the treating hospitals patient information sheets, consent forms, and datasheets. For patients who consent to participation, hospital investigators report by fax the demographic characteristics of the patients and information on the circumstances of the bite, its clinical effects, laboratory investigations, complications, and treatments. Missing data are extracted from hospital medical or pathology result records, as needed. All data are entered into a relational database (Microsoft Access) and are reviewed by the chief investigator (GKI). Blood samples are routinely collected from patients during their hospital stay and are frozen for subsequent laboratory analysis.
For this article, we included patients recruited during 1 January 2005 – 31 December 2019 for whom D‐dimer values within 24 hours of bites were recorded. We extracted information on the demographic characteristics of the patient, snake identification, envenoming‐related clinical syndromes (VICC, myotoxicity, neurotoxicity, acute kidney injury, thrombotic microangiopathy), and coagulation study results (INR, aPTT, fibrinogen and D‐dimer levels). We included only D‐dimer measurements from quantitative laboratory assays undertaken at the treating hospital (that is, not point‐of‐care D‐dimer test results). The snake species was determined by a licensed reptile handler or professional who worked with snakes, or by venom‐specific enzyme immunoassay of patient serum.
Systemic envenoming was determined according to defined clinical envenoming syndromes for Australian snakes, based on clinical features and laboratory testing.2 In our analysis, we separately considered two groups of patients: all patients with systemic envenoming, and patients with systemic envenoming and VICC (INR > 1.4 or fibrinogen < 2.0 mg/L). Patients who did not develop a clinical envenoming syndrome in hospital (within 12 hours of the snakebite) and did not later return to the hospital with delayed systemic envenoming were deemed to have not been envenomed.12 Acute kidney injury was defined according to the 2012 Kidney Disease: Improving Global Outcomes (KDIGO) guideline.15
Statistical analysis
Continuous variables are reported as medians with interquartile ranges (IQRs) and ranges. To estimate median changes in D‐dimer level, data were binned into 30‐minute periods from the snakebite, and D‐dimer value percentiles for each bin were plotted against median time, separately for non‐envenomed patients and patients with VICC. Each bin contained a median of 53 (IQR, 31–105) observations. The diagnostic performance of quantitative D‐dimer for diagnosing systemic envenoming with VICC was assessed as the area under the receiver operating characteristic curve (AUC) with its 95% confidence interval (CI). The optimal D‐dimer cut‐off value was defined as the value with the maximum sum of sensitivity and specificity (Youden index). All analyses were undertaken in GraphPad Prism for Windows 8.2.
Ethics approval
The human research ethics committees of the Northern Territory Department of Health and Menzies School of Health Research (04/08), the Hunter New England, the Royal Perth Hospital and South Metro Area Health Service (RA‐08/003), the Western Australian Country Health Service (2008: 03, REC200835), the Tasmania Network (H00109965), and the Gold Coast Health Service District (200835) approved the study.
Results
D‐dimer values within 24 hours of snakebite were recorded for 1363 of 2077 patients recruited to the ASP during 2005–2019 (66%; excluded: no quantitative D‐dimer testing, 652 patients; quantitative D‐dimer testing later than 24 hours, 62 patients, including 20 who presented more than 24 hours after the bite). The median age of included patients was 39 years (IQR, 22–55 years; range, 1–92 years); 970 were male (71%). Systemic envenoming was diagnosed in 790 included patients (58%): 364 with complete VICC (27%), 208 with partial VICC (15%), and 218 without VICC (16%). Myotoxicity (with or without VICC) developed in 125 patients (16%), neurotoxicity in 77 (10%). The snakes most frequently identified in envenoming cases were brown snakes (Pseudonaja spp.) (214 cases, 27%). Basic demographic and case characteristics were similar for the 1363 snakebite cases with D‐dimer testing within 24 hours and the 652 cases without recorded D‐dimer results (Box 1).
D‐dimer measurements within six hours of snakebite
D‐dimer values within six hours of snakebite were available for 1112 patients (82%; 102 of the 251 people for whom they were not available [41%] had presented more than six hours after the bite). For the 437 patients with VICC (full or partial), the 25th percentile value was greater than 2.5 mg/L within one hour of the bite (that is, the D‐dimer level was higher than 2.5 mg/L in 75% of patients), and by 2.8 hours the 5th percentile value was 2.8 mg/L (that is, the D‐dimer level was higher in 95% of patients). For 507 non‐envenomed patients, the 95th percentile D‐dimer value was less than 2.5 mg/L throughout the six hours following snakebite (that is, the D‐dimer level reached 2.5 mg/L in fewer than 5% of non‐envenomed patients) (Box 2).
The AUC for diagnosing envenoming on the basis of quantitative D‐dimer testing within six hours of snakebite was 0.87 (95% CI, 0.85–0.89). If patients with envenoming but not VICC were excluded, the AUC was 0.97 (95% CI, 0.96–0.98). For patients with VICC, the AUC, sensitivity, and specificity increased with time during the first three hours after the bite (to AUC, 1.0; sensitivity, 99%; specificity, 100%). For D‐dimer values measured 2–6 hours after the bite, the AUC was 0.99 (95% CI, 0.99–1.0); a cut‐point of 2.51 mg/L achieved 97.1% (95% CI, 95.0–98.3%) sensitivity and 99.0% (95% CI, 97.6–99.6%) specificity for diagnosing VICC. AUC, optimal cut‐point, sensitivity, and specificity for measurements 1–6 hours after the bite were similar to those for measurements 2–6 hours after the bite (Box 3; Supporting Information).
D‐dimer measurements for patients with VICC but normal INR and aPTT values
D‐dimer values were recorded within six hours of snakebite for 93 patients with VICC but normal INR (< 1.4) and aPTT values (< 40 seconds) on admission (median time to D‐dimer measurement, 1.25 hours; IQR, 0.67–1.83 hours; range: 0.25–6 hours). The AUC for diagnosing envenoming with VICC on the basis of D‐dimer value within six hours was 0.86 (95% CI, 0.81–0.91). For the 36 patients with D‐dimer values measured 2–6 hours after the bite, the AUC was 0.97 (95% CI, 0.93–1.0); with a cut‐point of 1.4 mg/L, sensitivity was 94% (95% CI, 82–99%) and specificity 96% (95% CI, 94–97%).
Abnormal INR or aPTT values were subsequently recorded for 65 of the 93 patients. INR and aPTT values remained within the normal ranges for 28 patients, who had low fibrinogen levels (< 2.0 g/L) indicating partial VICC; none experienced major haemorrhage, thrombotic microangiopathy, or acute kidney injury, while neurotoxicity and myotoxicity developed in two patients (both: taipan bites), myotoxicity alone in two, and neurotoxicity alone in one. Initial D‐dimer values exceeded 1.4 mg/L in 27 of the 28 patients; for one patient who experienced mild neurotoxicity, the D‐dimer level was 0.22 mg/L at 45 minutes and 6.73 mg/L at 7.25 hours.
D‐dimer values in patients who developed complications
D‐dimer values from within 24 hours of snakebite were available for 84 patients who developed thrombotic microangiopathy or acute kidney injury related to VICC. The D‐dimer concentration exceeded 4 mg/L in 83 patients (median, 20 mg/L; IQR, 20–32 mg/L) (Box 4). For one patient, the D‐dimer concentration 1.3 hours after the bite was 0.23 mg/L, when INR and aPTT values were normal; nine hours after the bite, the INR was 1.7 and the aPTT 100 seconds, and the patient developed acute kidney injury stage 2. Their D‐dimer level was not re‐assayed.
Discussion
We found in our large study of patients with confirmed or suspected snakebite that an elevated D‐dimer value within six hours of the bite was diagnostic of envenoming with VICC. The optimal cut‐off value was 2.5 mg/L; diagnostic accuracy increased across the first three hours after the bite. The optimal cut‐off value for patients with VICC but initially normal INR/aPTT values was 1.4 mg/L; diagnostic accuracy was greatest for tests 2–6 hours after the bite. In almost all patients who developed thrombotic microangiopathy or acute kidney injury associated with VICC, D‐dimer values exceeded 4 mg/L within 24 hours of the bite.
Within 2–3 hours of snakebite, changes in D‐dimer level clearly distinguished between non‐envenomed patients and patients with VICC (Box 2). During the first two hours, the D‐dimer concentration was lower than 2.5 mg/L in some patients who subsequently developed VICC, indicating that it should be re‐assessed later if the initial value is below this cut‐off. Test sensitivity increased during the first three hours, while specificity reached 94% in the first hour (Box 3). These findings suggest that a D‐dimer value greater than 2.5 mg/L within two hours of snakebite is an indication for antivenom, but the measurement should be repeated as soon as possible after two hours if the initial value is below 2.5 mg/L.
As most patients who develop VICC have abnormal INR/aPTT values on admission, quantifying D‐dimer would be most useful for those who initially present with normal INR/aPTT values but later develop VICC. Blood is first collected from many patients less than two hours after the bite, when the D‐dimer level was more likely to be below 2.5 mg/L. In 36 patients with normal INR/aPTT results, D‐dimer testing 2–6 hours after the bite was 94% sensitive and 96% specific for diagnosing VICC with a cut‐off of 1.4 mg/L, and this lower cut‐off may be useful for diagnosing patients for whom initial INR/PTT results are normal. However, complications for the 28 patients with persistently normal INR/aPTT results were mild.
D‐dimer values for patients who developed acute kidney injury or thrombotic microangiopathy associated with VICC exceeded 4 mg/L 2–24 hours after the bite. This may help early recognition of patients at risk of these complications, although they can also be identified by elevated creatinine levels or schistocytosis on a blood film.
Our findings cannot be directly extrapolated to patients with bites from some other groups of snakes that also cause VICC, such as vipers.16,17 D‐dimer levels reflect the breakdown products of cross‐linked fibrin (cross‐linked degradation products, XDPs), produced only when the clotting pathway is normally activated and fibrinogen is converted to fibrin and cross‐linked by factor XIII. The venom of many pit vipers (Crotalinae) contains thrombin‐like enzymes that consume fibrinogen without producing normal fibrin for cross‐linking.18 Consequently, most breakdown products are fibrinogen degradation products (FDPs), not the XDPs detected by the D‐dimer assay. Clinical studies of bites by snakes from different viper groups could determine whether D‐dimer level is diagnostic in these cases.
Clinical translation of our findings would require the availability of D‐dimer testing in the smaller and rural hospitals to which many people with snakebite initially present. Point‐of‐care D‐dimer testing has been used for many years, but its usefulness for diagnosing VICC has not been evaluated. Point‐of‐care INR testing for diagnosing VICC after snakebite is not reliable,19 potentially making point‐of‐care D‐dimer testing even more valuable. However, D‐dimer testing will not be useful for diagnosing envenoming without VICC; for instance, following bites by black snakes (Pseudechis spp., including red‐bellied black snakes) or death adders (Acanthophis spp.). In these cases, non‐specific symptoms may be the only early indications of envenoming.
Limitations
A major limitation of our study was that D‐dimer testing was not undertaken within 24 hours for about one‐third of patients with suspected snakebite, but the demographic and envenoming characteristics of patients who were or were not tested were similar. Further, 22% of all D‐dimer assays were not conducted within six hours of snakebite (6–24 hours, 18%; later than 24 hours, 4%), in about 40% of cases because people presented to hospital more than six hours after being bitten. Interpreting and extrapolating our findings are also limited by variation in D‐dimer testing between laboratories with respect to instruments, reagents, and units of reporting. Quantitative D‐dimer results can be reported in D‐dimer units (DDU) or fibrinogen equivalent units (FEU; 1.0 mg/L FEU ≈ 0.5 mg/L DDU); the units employed (ng, mg, g per μL, mL, L) also differ by laboratory.20,21 We used mg/L as the standard reporting unit and excluded qualitative and semi‐quantitative methods of measurement. Our cut‐points are most directly applicable to D‐dimer results reported in FEU, the unit used by most Australian laboratories.21 Finally, many laboratories apply relatively low upper limits (4 or 20 mg/L) and report results only beyond this threshold; as a result, a large proportion of values were higher than 4 or 20 mg/L (Box 4).
Conclusion
Assessing D‐dimer level could be useful for the early diagnosis of systemic envenoming with VICC after snakebite. Assaying D‐dimer as soon as possible but at least two hours after the bite, with a cut‐off value of 2.5 mg/L, would provide high sensitivity and specificity, and allow sufficient time for administering antivenom within three hours of the snakebite.
Box 1 – Characteristics of the 1363 patients with recorded D‐dimer values (within 24 hours of snakebite) and of the 652 patients without recorded D‐dimer values
|
D‐dimer value within 24 hours of bite |
||||||||||||||
Characteristic |
Yes |
No* |
|||||||||||||
|
|||||||||||||||
Patients |
1363 |
652 |
|||||||||||||
Age (years), median (IQR) |
39 (22–55) |
38 (24–54) |
|||||||||||||
Sex (male) |
970 (70%) |
494 (76%) |
|||||||||||||
Snake type: definite identification |
676 (50%) |
422 (65%) |
|||||||||||||
Brown snake |
214 (16%) |
132 (20%) |
|||||||||||||
Red‐bellied black snake |
132 (10%) |
92 (14%) |
|||||||||||||
Tiger snake |
108 (8%) |
49 (8%) |
|||||||||||||
Systemic envenoming |
790 (58%) |
357 (55%) |
|||||||||||||
Venom‐induced consumption coagulopathy |
572 (42%) |
251 (38%) |
|||||||||||||
Myotoxicity |
125 (9%) |
47 (7%) |
|||||||||||||
Neurotoxicity |
77 (6%) |
32 (5%) |
|||||||||||||
Antivenom administered |
689 (51%) |
306 (47%) |
|||||||||||||
Hospital length of stay (hours), median (IQR) |
23 (17–44) |
23 (16–41) |
|||||||||||||
|
|||||||||||||||
IQR = interquartile range. * Excludes 62 patients with D‐dimer assays more than 24 hours after snakebite, including 20 who presented to hospital more than 24 hours after the snakebite. |
Box 2 – D‐dimer levels following snakebite for 437 envenomed patients with venom‐induced consumption coagulopathy (VICC; 838 measurements) and 507 non‐envenomed patients (968 measurements)*
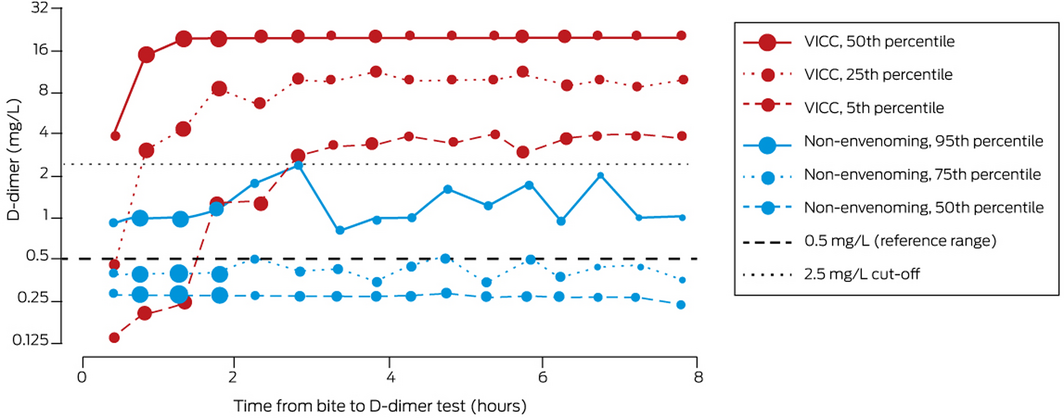
* Size of data points reflects number of measurements for time point: small, 26–50; medium, 51–75; large, 76–100; very large, 101–125. ◆
Box 3 – Area under the receiver operating characteristic curve (AUC), optimal cut‐point, sensitivity, and specificity for diagnosis of venom‐induced consumption coagulopathy, based on D‐dimer measurement within six hours of bite, by time since bite
Time (hours) |
Measurements |
AUC (95% CI) |
Cut‐point (mg/L) |
Sensitivity |
Specificity |
||||||||||
|
|||||||||||||||
0–1 |
248 |
0.90 |
0.75 |
78.9% |
94.3% |
||||||||||
1–2 |
357 |
0.97 |
1.44 |
91.4% |
97.9% |
||||||||||
2–3 |
216 |
0.99 |
2.80 |
94.6% |
100% |
||||||||||
3–4 |
237 |
1.0 |
1.59 |
99.0% |
100% |
||||||||||
4–5 |
204 |
1.0 |
2.98 |
98.8% |
100% |
||||||||||
5–6 |
203 |
1.0 |
2.40 |
98.8% |
99.2% |
||||||||||
1–6 |
705 |
0.99 |
2.51 |
94.7% |
99.1% |
||||||||||
2–6 |
381 |
0.99 |
2.51 |
97.1% |
99.0% |
||||||||||
|
|||||||||||||||
|
Box 4 – D‐dimer level for 84 patients who developed thrombotic microangiopathy or acute kidney injury related to venom‐induced consumption coagulopathy*
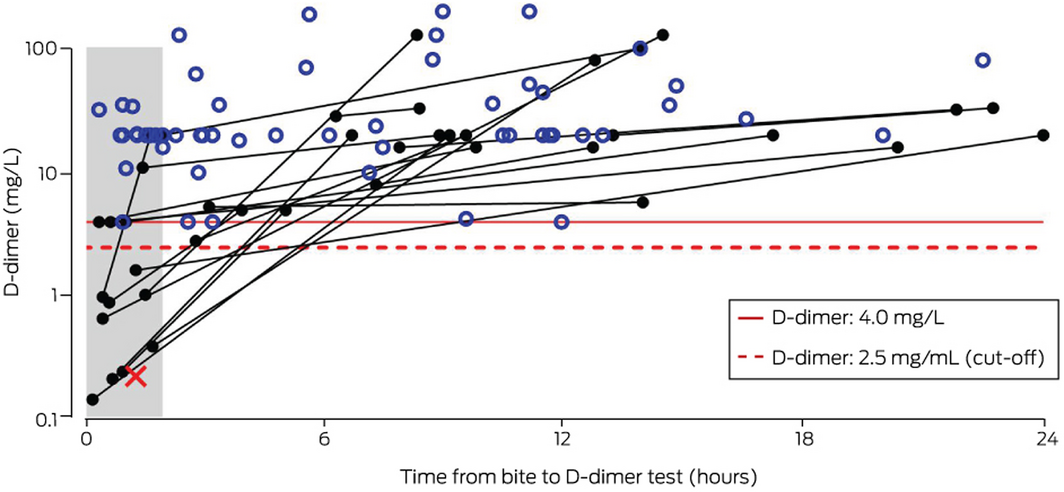
* For 61 patients, the initial and maximum D‐dimer values were identical and greater than 4 mg/L (open blue circles); for 22 patients, the maximum value was greater than the first measurement and greater than 4 mg/L (filled black circles connected by black lines); for one patient, the initial D‐dimer value was below 2.5 mg/L and the test was not repeated (red cross). ◆
Received 29 December 2021, accepted 16 March 2022
- Geoffrey K Isbister1,2
- Tina Noutsos3
- Shane Jenkins1
- Katherine Z Isoardi4,5
- Jessamine Soderstrom6,7
- Nicholas A Buckley8
- 1 The University of Newcastle, Newcastle, NSW
- 2 Calvary Mater Newcastle, Newcastle, NSW
- 3 Menzies School of Health Research, Darwin, NT
- 4 Princess Alexandra Hospital, Brisbane, QLD
- 5 Queensland Poisons Information Centre, Queensland Children’s Hospital, Brisbane, QLD
- 6 Royal Perth Hospital, Perth, WA
- 7 Western Australia Poisons Information Centre, Sir Charles Gairdner Hospital, Perth, WA
- 8 The University of Sydney, Sydney, NSW
Open Access
Open access publishing facilitated by The University of Newcastle, as part of the Wiley ‐ The University of Newcastle agreement via the Council of Australian University Librarians.
Geoffrey Isbister is supported by a National Health and Medical Research Council (NHMRC) Senior Research Fellowship (1154503). The study was funded by an NHMRC Centre for Research Excellence Grant (1110343). We acknowledge the assistance of the Australian Snakebite Project Research database administrators, including Kylie Tape (University of Newcastle; The Mater Hospital, Newcastle) and the local co‐investigators, and the medical, nursing, and laboratory staff at referring centres around Australia who assisted in patient recruitment and data collation.
No relevant disclosures.
- 1. Kasturiratne A, Wickremasinghe AR, de Silva N, et al. The global burden of snakebite: a literature analysis and modelling based on regional estimates of envenoming and deaths. PLoS Med 2008; 5: e218.
- 2. Johnston CI, Ryan NM, Page CB, et al. The Australian Snakebite Project, 2005–2015 (ASP‐20). Med J Aust 2017; 207: 119‐125. https://www.mja.com.au/journal/2017/207/3/australian‐snakebite‐project‐2005‐2015‐asp‐20
- 3. Noutsos T, Currie BJ, Isoardi KZ, et al. Snakebite‐associated thrombotic microangiopathy: an Australian prospective cohort study (ASP30). Clin Toxicol (Phila) 2022; 60: 205‐213.
- 4. Sutherland SK. Deaths from snake bite in Australia, 1981–1991. Med J Aust 1992; 157: 740‐746.
- 5. Sutherland SK, Leonard RL. Snakebite deaths in Australia 1992–1994 and a management update. Med J Aust 1995; 163: 616‐618.
- 6. Silva A, Isbister GK. Current research into snake antivenoms, their mechanisms of action and applications. Biochem Soc Trans 2020; 48: 537‐546.
- 7. Johnston CI, Ryan NM, O’Leary MA, et al. Australian taipan (Oxyuranus spp.) envenoming: clinical effects and potential benefits of early antivenom therapy. Australian Snakebite Project (ASP‐25). Clin Toxicol (Phila) 2017; 55: 115‐122.
- 8. Johnston CI, Brown SG, O’Leary MA, et al; ASP investigators. Mulga snake (Pseudechis australis) envenoming: a spectrum of myotoxicity, anticoagulant coagulopathy, haemolysis and the role of early antivenom therapy. Australian Snakebite Project (ASP‐19). Clin Toxicol (Phila) 2013; 51: 417‐424.
- 9. Sanhajariya S, Duffull SB, Isbister GK. Population pharmacokinetics of Pseudechis porphyriacus (red‐bellied black snake) venom in snakebite patients. Clin Toxicol (Phila) 2021; 59: 956‐962.
- 10. Trevett AJ, Lalloo DG, Nwokolo NC, et al. The efficacy of antivenom in the treatment of bites by the Papuan taipan (Oxyuranus scutellatus canni). Trans R Soc Trop Med Hyg 1995; 89: 322‐325.
- 11. Churchman A, O’Leary MA, Buckley NA, et al. Clinical effects of red‐bellied black snake (Pseudechis porphyriacus) envenoming and correlation with venom concentrations. Australian Snakebite Project (ASP‐11). Med J Aust 2010; 193: 696‐700. https://www.mja.com.au/journal/2010/193/11/clinical‐effects‐red‐bellied‐black‐snake‐pseudechis‐porphyriacus‐envenoming‐and
- 12. Ireland G, Brown SG, Buckley NA, et al. Changes in serial laboratory test results in snakebite patients: when can we safely exclude envenoming? Med J Aust 2010; 193: 285‐290. https://www.mja.com.au/journal/2010/193/5/changes‐serial‐laboratory‐test‐results‐snakebite‐patients‐when‐can‐we‐safely
- 13. Gulati A, Isbister GK, Duffull SB. Effect of Australian elapid venoms on blood coagulation: Australian Snakebite Project (ASP‐17). Toxicon 2013; 61: 94‐104.
- 14. Patel P, Patel P, Bhatt M, et al. Systematic review and meta‐analysis of test accuracy for the diagnosis of suspected pulmonary embolism. Blood Adv 2020; 4: 4296‐4311.
- 15. Kidney Disease: Improving Global Outcomes acute kidney injury working group. KDIGO clinical practice guideline for acute kidney injury. Kidney Int Suppl 2012; 2: 1‐138.
- 16. Mion G, Larréché S, Benois A, et al. Hemostasis dynamics during coagulopathy resulting from Echis envenomation. Toxicon 2013; 76: 103‐109.
- 17. Isbister GK, Maduwage K, Scorgie FE, et al. Venom concentrations and clotting factor levels in a prospective cohort of Russell’s viper bites with coagulopathy. PLoS Negl Trop Dis 2015; 9: e0003968.
- 18. Sano‐Martins IS, Fan HW, Castro SC, et al; Butantan Institute Antivenom Study Group. Reliability of the simple 20 minute whole blood clotting test (WBCT20) as an indicator of low plasma fibrinogen concentration in patients envenomed by Bothrops snakes. Toxicon 1994; 32: 1045‐1050.
- 19. O’Rourke KM, Correlje E, Martin CL, et al. Point‐of‐care derived INR does not reliably detect significant coagulopathy following Australian snakebite. Thromb Res 2013; 132: 610‐613.
- 20. Longstaff C, Adcock D, Olson JD, et al. Harmonisation of D‐dimer: a call for action. Thromb Res 2016; 137: 219‐220.
- 21. Favaloro EJ, Dean E. Variability in D‐dimer reporting revisited. Pathology 2021; 53: 538‐540.
Abstract
Objective: To assess the accuracy and marginal value of quantitative D‐dimer testing for diagnosing venom‐induced consumption coagulopathy (VICC) in people bitten by Australian snakes.
Design, setting: Analysis of data for suspected and confirmed cases of snakebite collected prospectively by the Australian Snakebite Project, 2005–2019, from 200 hospitals across Australia.
Participants: 1363 patients for whom D‐dimer was quantitatively assessed within 24 hours of suspected or confirmed snakebite.
Main outcome measures: Diagnostic performance of quantitative D‐dimer testing for detecting systemic envenoming with VICC (area under the receiver operating characteristic curve, AUC); optimal D‐dimer cut‐off value (maximum sum of sensitivity and specificity).
Results: D‐dimer values exceeded 2.5 mg/L within three hours of the bite for 95% of patients who developed VICC, and were lower than 2.5 mg/L for 95% of non‐envenomed patients up to six hours after snakebite. The AUC for diagnosing envenoming with VICC on the basis of quantitative D‐dimer testing within six hours of snakebite was 0.97 (95% CI, 0.96–0.98; 944 patients). Diagnostic performance increased during the first three hours after snakebite; for quantitative D‐dimer testing at 2–6 hours, the AUC was 0.99 (95% CI, 0.99–1.0); with a cut‐off of 2.5 mg/L, sensitivity was 97.1% (95% CI, 95.0–98.3%) and specificity 99.0% (95% CI, 97.6–99.6%) for VICC. For 36 patients with normal international normalised ratio (INR) and activated partial thromboplastin time (aPTT) values 2–6 hours after snakebite, the AUC was 0.97 (95% CI, 0.93–1.0); with a cut‐off of 1.4 mg/L, sensitivity was 94% (95% CI, 82–99%) and specificity 96% (95% CI, 94–97%). In all but one of 84 patients who developed VICC‐related acute kidney injury, D‐dimer values exceeded 4 mg/L within 24 hours of the bite.
Conclusion: D‐dimer concentrations assessed 2–6 hours after snakebite, with a cut‐off value of 2.5 mg/L, could be useful for diagnosing envenoming with VICC.