In Australia, prostate cancer is, after skin cancer, the most common cancer diagnosed, and it is second only to lung cancer as a cause of cancer-related deaths.1-4 In 2005, prostate cancer was the most prevalent cancer for Australian men, accounting for over 29% of all cancer diagnoses.2
In EBRT for prostate cancer, high-energy x-ray beams from multiple directions deposit energy (dose) within the tumour (prostate) to destroy cancer cells. The standard process for EBRT treatment planning is shown in Box 1(A). The first step is patient imaging (a CT scan sometimes combined with MRI). The treatment targets (the prostate and sometimes the seminal vesicles) and important normal tissues (the rectum, bladder, and femoral heads) are then manually defined from the scans (Box 2). Modern radiotherapy machines offer improved treatment accuracy through better visualisation and the correction of errors in patient setup, making target delineation the most significant uncertainty in radiotherapy planning.
MRI scans have vastly superior soft-tissue contrast compared with CT scans, with better visualisation and more accurate delineation of the border of the prostate. Prostate borders delineated on MRI scans show greater consistency between radiation oncologists,5,6 and prostate size is smaller than that determined by CT scan.7 Using MRI, the size of the extra margins to be added to account for delineation uncertainties is reduced, and less normal tissue is irradiated, thus reducing treatment toxicity.
A proposed new workflow for prostate radiation therapy planning using MRI scans only is shown in Box 1(B). The prostate and organs would be automatically defined on a high-contrast MRI scan using computer segmentation (organ delineation) algorithms. These algorithms use an “average” MRI scan that has been created from a set of patient MRI scans, commonly known as an “atlas”. This atlas is deformably “warped” (registered) to the patient’s MRI scan until all the tissues line up with the patient’s MRI scan. As the organ boundaries are known in the MRI atlas, these boundaries are also warped onto the patient’s MRI scan and hence the patient’s organ boundaries are determined.
Probabilistic atlas-based organ segmentation methods were developed to automatically segment the organs of interest (prostate, rectum, bladder, and femoral heads) from the MRI scans. After the scans were preprocessed (including correction of intensity inhomogeneity8 and patient movement artefacts9), the images and manually defined contours for each patient were combined into a single reference atlas by image registration methods. This atlas can then be warped onto the MRI scans of a new patient, automatically contouring the organs of interest (Box 3). A matching CT atlas enables the automatic estimation of electron densities from MRI scans by the same method.
First, for each patient and each organ of interest, a comparison was made between the Hounsfield units (HUs) (a quantitative measure of electron density needed to calculate radiation attenuation) of the pseudo-CT scan and the HUs of the original CT scan.
Second, for each patient, a ratio was calculated between the lateral treatment beam physical path length (distance from the skin to the tumour dose calculation point) and the water equivalent path length (distance from the skin to the same calculation point) on both the CT and pseudo-CT scans.
Another important part of the new workflow process is the production of customised software that has been written and validated (i) for information transfer from the Varian Eclipse and Philips Pinnacle treatment planning systems at Calvary Mater Newcastle (Digital Imaging and Communications in Medicine – Radiotherapy [DICOM-RT] and Radiation Therapy Oncology Group [RTOG]),10 and (ii) for transfer of automatically defined contours and pseudo-CT images back into each system for dose planning.
As shown in Box 4, for the main organs of interest for the 39 patients, HU values of the generated pseudo-CT scans and the original CT scans were very similar, with no significant differences observed (P > 0.09). The mean ratios (SDs) of physical path length and water equivalent path length for the left lateral beams were 1.068 (0.02) and 1.089 (0.02) (P < 0.001), and for the right lateral beams were 1.074 (0.02) and 1.084 (0.03) (P = 0.09). These results indicate that pseudo-CT scan HU values approximate CT scan HU values well, particularly for bone. A significant difference observed for the left lateral beams is not enough to result in dose-calculation differences.
1 (A) Steps in the conventional prostate radiotherapy workflow with computed tomography (CT) and magnetic resonance imaging (MRI); and (B) the proposed new radiotherapy workflow based on MRI only
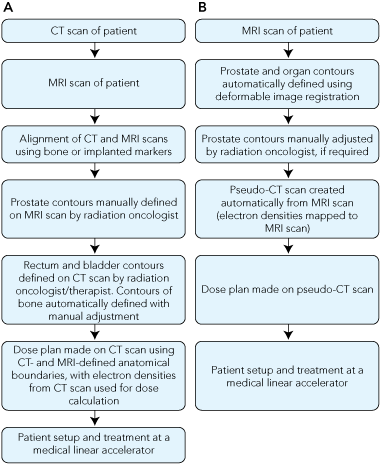
2 Conventional computed tomography scans for delineating the prostate and surrounding normal tissues, and calculating radiation dose
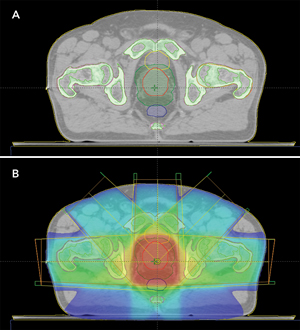
3 Automatic magnetic resonance image (MRI) segmentation results
![]() |
![]() |
A: Axial, coronal and sagittal views of the MRI scan of the pelvic region of one patient. |
B: Expert organ contours manually delineated from the scan in A (bones, bladder, rectum and prostate). |
![]() |
![]() |
D: A surface rendering of the organs in the MRI “atlas” used to perform the automatic segmentation. |
- Peter B Greer1,2
- Jason A Dowling3
- Jonathon A Lambert2
- Jurgen Fripp3
- Joel Parker1
- James W Denham1,2
- Chris Wratten1,2
- Anne Capp1,2
- Olivier Salvado3
- 1 Calvary Mater Newcastle Hospital, Newcastle, NSW.
- 2 University of Newcastle, Newcastle, NSW.
- 3 Biomedical Imaging, Australian e-Health Research Centre, CSIRO ICT Centre, Brisbane, QLD.
This work was partially funded by Cancer Council NSW, Project Grant RG 07-06. We gratefully acknowledge the expert assistance received during this project from: Jacqueline Patterson and Dr Colin Tang (Calvary Mater Newcastle Hospital, Newcastle); Dr Peter Lau, Kristen Fisher and Cynthia Hughes (Hunter Imaging Group, Maitland Private Hospital, Newcastle); Dr Pierrick Bourgeat (CSIRO Australian e-Health Research Centre); Dr Martin Ebert (Department of Radiation Oncology, Sir Charles Gairdner Hospital, Perth); and Dr Sebastien Ourselin (Centre for Medical Image Computing, University College London, UK).
None identified.
- 1. Australian Institute of Health and Welfare. Australian cancer incidence and mortality (ACIM) books. Canberra: AIHW, 2007. http://www.aihw.gov.au/cancer/data/acim_books/ (accessed Nov 2010).
- 2. Australian Institute of Health and Welfare and Australasian Association of Cancer Registries. Cancer in Australia: an overview, 2008. Canberra: AIHW/AACR, 2008. (Cancer Series No. 46; Cat. No. CAN 42.) http://www.aihw.gov.au/publications/can/ca08/ca08.pdf (accessed Nov 2010).
- 3. Lavelle P. Prostate cancer [online article]. ABC Health and Wellbeing. 2003 (last updated 19 Jan 2007). http://www.abc.net.au/health/library/stories/2003/05/15/1831519.htm (accessed Nov 2010).
- 4. National Health and Medical Research Council. Clinical practice guidelines: evidence-based information and recommendations for the management of localised prostate cancer. Canberra: NHMRC, 2002. http://www.nhmrc.gov.au/_files_nhmrc/file/publications/synopses/cp88.pdf (accessed Nov 2010).
- 5. Roach M 3rd, Faillace-Akazawa P, Malfatti C, et al. Prostate volumes defined by magnetic resonance imaging and computerized tomographic scans for three dimensional conformal radiotherapy. Int J Radiat Oncol Biol Phys 1996; 35: 1011-1018.
- 6. Debois M, Oyen R, Maes F, et al. The contribution of magnetic resonance imaging to the three-dimensional treatment planning of localized prostate cancer. Int J Radiat Oncol Biol Phys 1999; 45: 857-865.
- 7. Rasch C, Barillot I, Remeijer P, et al. Definition of the prostate in CT and MRI: a multi-observer study. Int J Radiat Oncol Biol Phys 1999; 43: 57-66.
- 8. Dowling J, Bourgeat P, Raffelt D, et al. Non-rigid correction of interleaving artefacts in pelvic MRI. In: Pluim JPW, Dawant BM, editors. Proceedings of SPIE Medical Imaging 2009: Image Processing (Proceedings Volume 7259, Article No. 72592P).
- 9. Salvado O, Hillenbrand C, Zhang S, Wilson DL. Method to correct intensity inhomogeneity in MR images for atherosclerosis characterization. IEEE Trans Med Imaging 2006; 25: 539-552.
- 10. Dowling J, Malaterre M, Greer PB, Salvado O. Importing contours from DICOM-RT structure sets. The Insight Journal 2009; Jul–Dec. http://www.insight-journal.org/browse/publication/701 (accessed Nov 2010).
Abstract
Dose planning for prostate radiation therapy is performed using computed tomography (CT) scans that provide the electron density information needed for individual patients’ radiation dose calculations.
For visualising the prostate and determining the target volume for radiation treatment, magnetic resonance imaging (MRI) gives vastly superior soft-tissue contrast. However, currently, MRI scans cannot be used for dose planning, as they do not provide the electron density information.
We aimed to develop an alternative and efficient MRI-only image-based workflow, enabling both organ delineation and dose planning to be performed using MRI, with “pseudo-CT scans” generated from MRI scans supplying the information for dose planning.
The feasibility of implementing MRI-based prostate radiation therapy planning is being investigated through collaboration between the clinical and medical physics group at the Calvary Mater Newcastle Hospital/University of Newcastle and the biomedical imaging processing group at the CSIRO (Commonwealth Scientific and Industrial Research Organisation) Australian e-Health Research Centre.
Results comparing Hounsfield units calculated from CT scans and from MRI-based pseudo-CT scans for 39 patients showed very similar average values for the prostate, bladder, bones and rectum, confirming that pseudo-CT scans can replace CT scans for accurate radiation dose calculations.
MRI-based radiotherapy planning can also be used for tumours in other locations, such as head and neck, and breast cancers.